Optimising Frequency Positioning of Tunable Couplers: Experimental Results from the DiCarlo Lab on QuantWare QPU
Abstract:
This article presents a summary of recent experimental work carried out by the DiCarlo Lab at QuTech as part of the Hectoqubit/2 initiative involving the Delft University of Technology, QuantWare, and partners such as Orange Quantum Systems.
The experiments were conducted on a 5-qubit prototype of QuantWare’s commercially available 17-qubit Contralto-A quantum processor. The study investigates how the frequency positioning of tunable couplers impacts crosstalk and spectator qubit effects across single-qubit gates, 2-qubit CZ gates, and readout operations. The results underscore the critical role tunable couplers play in realising scalable superconducting QPUs optimised for quantum error correction (QEC) and establish optimised methods for using them to achieve high-fidelity operations. The findings directly inform the design philosophy of Contralto-A, QuantWare’s QEC-dedicated QPU, and mark an important step toward the development of fault-tolerant, utility-scale quantum systems.
Tunable Couplers and the Drive Toward Scalable QEC
As the quantum computing industry progresses toward large-scale fault tolerance, tunable couplers have become a paramount enabler of scalable, high-fidelity quantum operations. Unlike fixed couplers, which provide a static, non-adjustable coupling strength between qubits, tunable couplers allow qubit-to-qubit interactions to be dynamically adjusted. This enables the selective suppression of residual qubit interactions (ZZ) or unwanted exchange couplings (J) which are common sources of crosstalk in multi-qubit systems.
This flexibility unlocks more precise gate operations and minimises crosstalk, which is a prerequisite for any QPU intended for error correction. However, tunable couplers also introduce new design complexity, including tradeoffs in how spectator qubit effects (residual interactions from inactive qubits) are mitigated during different classes of operations.
Experimental Focus and Methodology
The experiments at the DiCarlo lab are part of a growing body of research focused on understanding how to harness the potential of tunable couplers while managing the complex side effects they introduce, such as spectator qubit interactions and residual coupling.
This study marks the first set of experiments conducted on a QuantWare-built tunable coupler QPU. The 5-qubit device used in the study is a prototype version of our currently commercialised Contralto-A, QuantWare’s 17-qubit QPU with 24 tunable couplers, specifically designed for quantum error correction (QEC).
The researchers systematically investigated how coupler frequency positioning impacts crosstalk across three types of quantum operations: single-qubit gates, readout, two-qubit CZ gates.
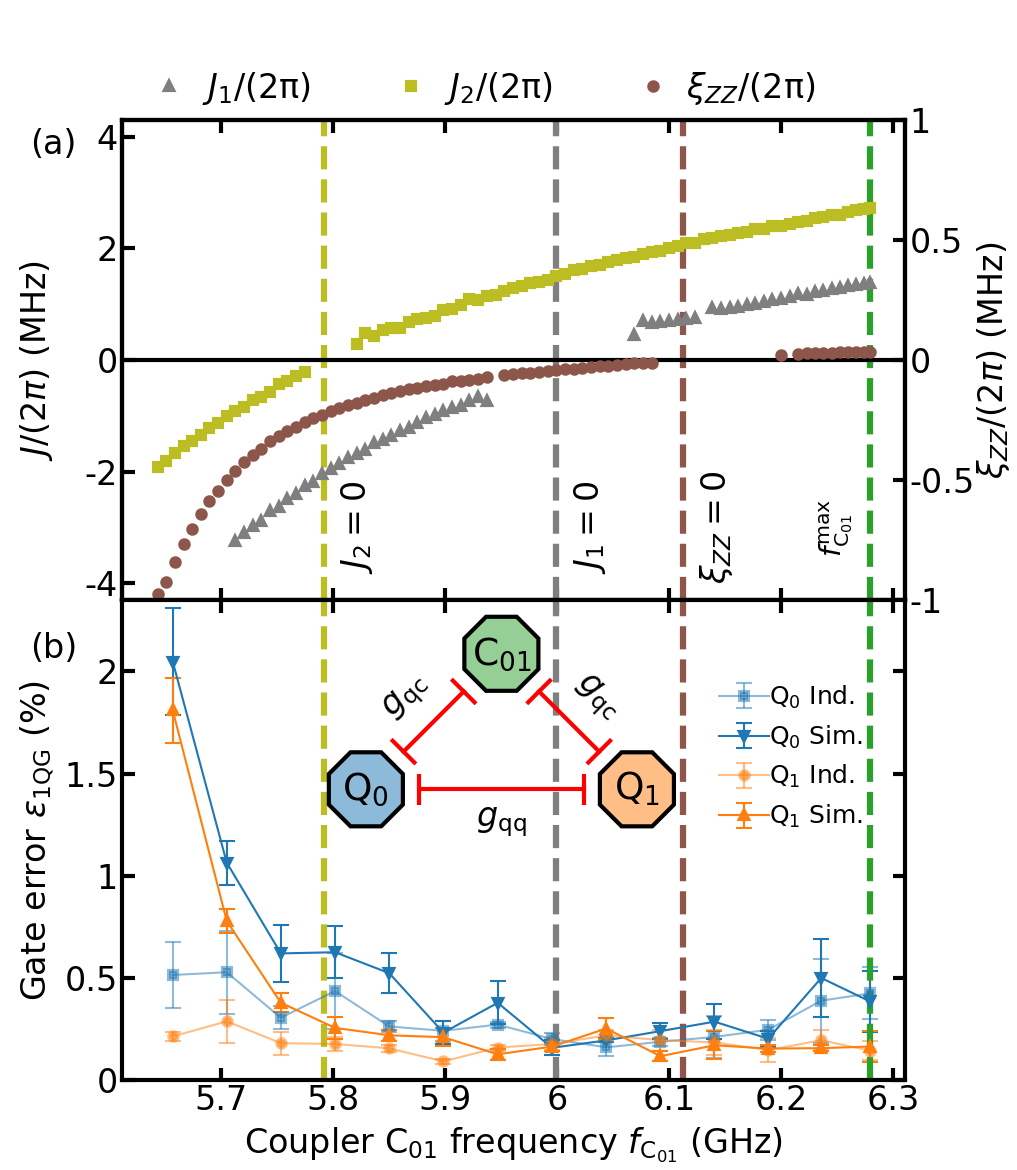
Single-qubit gates: Randomised benchmarking was used to track fidelity as a function of coupler frequency. A broad low-error region was observed near the ZZ = 0 point, with minima aligning with the suppression of exchange interaction J1.
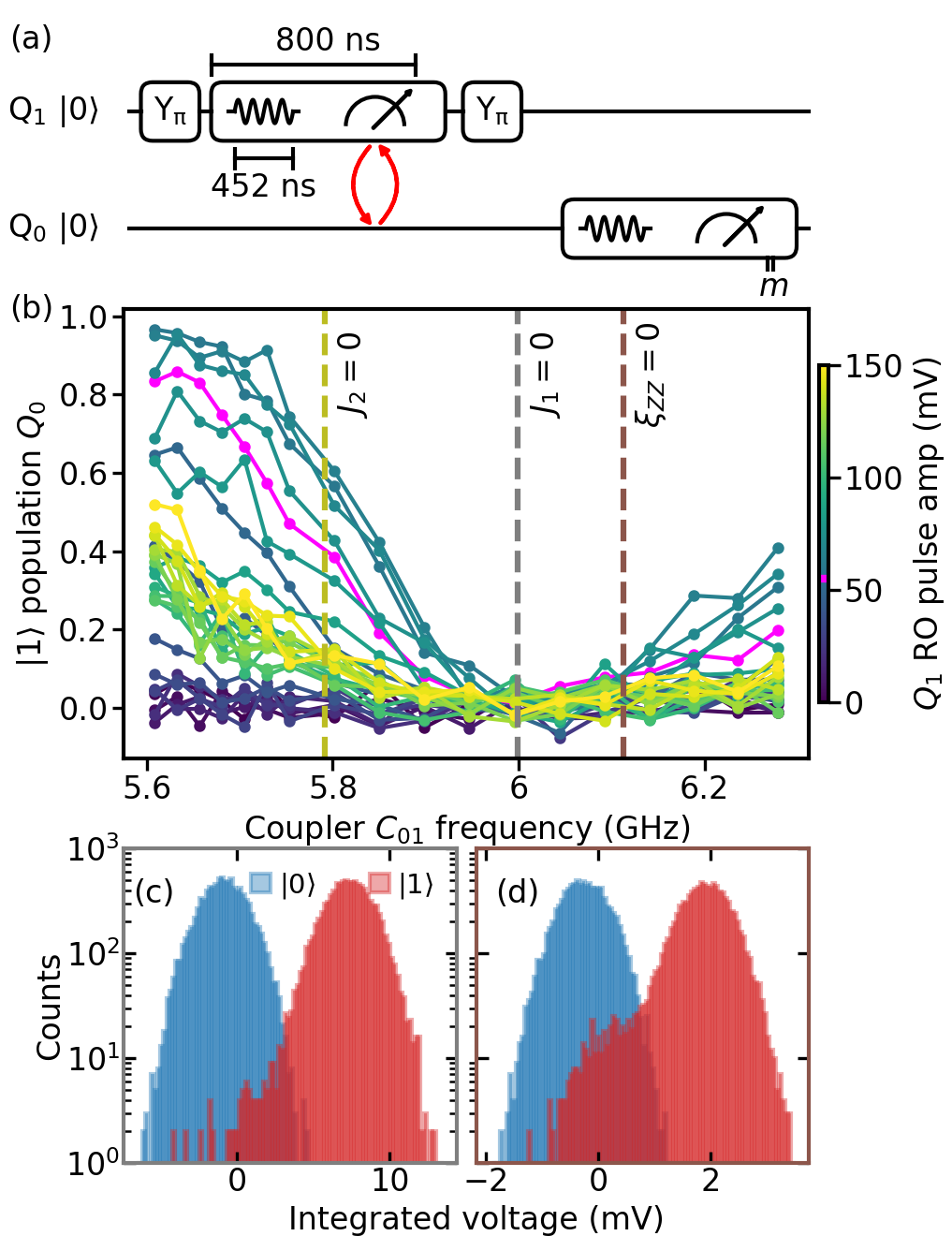
Readout: Residual J1 coupling at the coupler ZZ-offpoint led to population exchange between qubits during measurement. The experiments showed this could be nullified by turning J1 ≈ 0, significantly improving readout fidelity.

Two-qubit CZ gates: High-fidelity CZ gates (<1% error) were implemented using fast-adiabatic flux pulsing of the coupler only, while keeping qubits at their coherence sweetspots. Gate quality was maximised when residual ZZ interactions were suppressed.
To assess system-level performance, the team also implemented repeated weight-2 parity checks. Here, coupler positioning directly influenced the resulting defect rates, validating the need for dynamic tunability and careful calibration in QEC systems.
Tradeoff: While ZZ, J1 , and J2 interactions can each be individually nulled, they cannot all be simultaneously nulled at a single coupler frequency. The optimal configuration depends on the specific operation. The performance of QEC protocols is demonstrated by combining tunable couplers with an optimal configuration.
From Prototype to Large-Scale QEC

Contralto-A builds on the principles explored in this study. Designed by QEC experts, it features a ‘ninja star’ qubit layout, 17 high-coherence transmon qubits, and 24 tunable couplers, all optimised for running distance-3 surface code algorithms. The inclusion of Purcell filters and dedicated drive and flux lines further supports the integration, operation, and demonstration of high-fidelity operations and advanced quantum computing systems.
As the largest commercially available QPU dedicated to QEC development, Contralto-A provides a direct path from research-grade prototypes toward large-scale fault-tolerant systems.
Looking Ahead
This paper deepens the quantum field’s understanding of tunable coupler behaviour and emphasises their value in balancing coherence, connectivity, and controllability, fundamental demands of QEC systems. The results also validate the design architecture strategy behind Contralto-A, and by extension epitomise QuantWare’s vision for accelerating the advent of utility-scale quantum computing.
The study also opens the door to further investigations. As noted by the authors, applying these mitigation strategies to larger processors, such as the 17-qubit, 24-tunable coupler layout designed for running distance-3 surface code, will be a critical step. This architecture is precisely what Contralto-A was built to deliver. Continued experiments on this larger platform will enable even deeper insights into crosstalk management at scale and offer a rigorous proving ground for the next generation of tunable coupler strategies.
Moreover, alternative coupling schemes capable of simultaneously suppressing both ZZ and exchange interactions remain a promising direction, one that could reshape the landscape of high-fidelity, tunable coupler connectivity.
As superconducting QPU architectures continue to evolve, dynamic, tunable systems will be central to scaling. This study marked an important step on that path and a glimpse of what is coming next.
Read the full paper: https://arxiv.org/abs/2503.13225